Dustin Kleckner, Martin Scheeler, William T. M. Irvine
University of Chicago
It was nearly 150 years ago that Lord Kelvin first proposed his atomic theory in which knots in the Ether acted as the fundamental building blocks for matter. While the Ether was soon ushered out of style, knots have remained a fruitful mathematical and scientific interest for over a century, appearing in a wide range of topics, from quantum field theories to electromagnetism to plasmas and fluid flows [1-3]. Although knots in the vorticity field of a fluid have long been studied in theory [4], success in generating and observing these topological vortex loops in a real fluid has only recently been achieved [5].
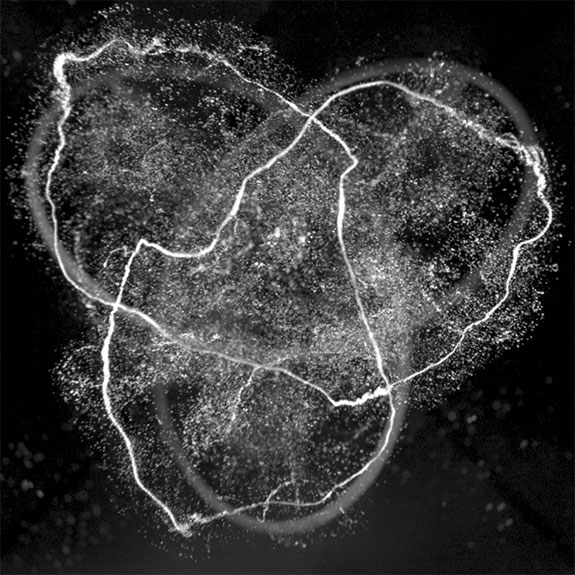
Figure 1 shows one such topological vortex loop tied into a trefoil knot evolving in water. The knot is generated in the presence of tiny micro-bubbles, which are pulled into the low-pressure region at the vortex core where they scatter light, illuminating the structure of the vortex loop. In experiment, high-speed laser scanning tomography is used to capture the three-dimensional structure of the trefoil knot as it evolves, revealing its dynamics as it stretches and deforms itself until it ultimately undergoes a series of reconnection events that untie the knot into two unlinked rings. Interestingly, we observe that a pair of linked vortex rings follows this same general framework over its evolution: an initial rapid stretching of the rings as they approach a series of reconnection events that ultimately unlink them.
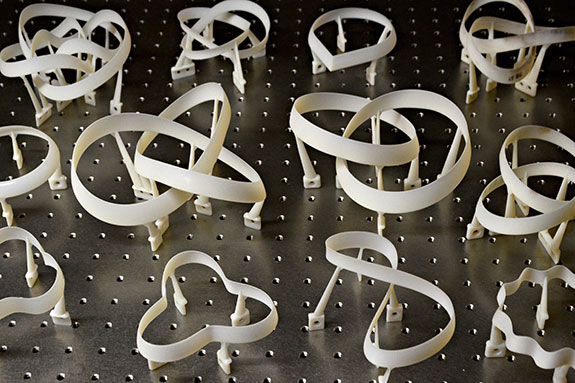
In order to create these topological vortex loops, a novel method was developed in which the vortex loops are shed from an accelerated hydrofoil, a collection of which are shown in Figure 2. With a wing profile cross-section, the hydrofoils have a trailing edge that traces out the desired path of the vortex loop, such that upon impulsive acceleration, they shed a starting vortex with the same geometry and topology as the hydrofoil itself. Advances in rapid prototyping and 3D printing have allowed us to quickly fabricate a zoo of hydrofoils of different shapes and topologies, which let us explore a wide variety of vortex dynamics from a geometric and topological perspective.
This work is supported by the National Science Foundation (NSF) Materials Research and engineering Centers (MRSEC) at the University of Chicago, and by the A. P. Sloan Foundation and the Packard Foundation.
Meeting Abstract (Event: 203835)
Meeting Abstract (Event: 203612)
Usage Information
Reporters may freely use this image. Credit Dustin Kleckner and William T.M. Irvine /U.Chicago.
Resources
[1] Witten, E., “Quantum-field theory and the Jones polynomial”, Commun. Math Phys., 121, 351-399 (1989). URL: http://link.springer.com/article/10.1007%2FBF01217730
[2] Kedia et al., “Tying knots in light fields”, PRL 111, 150404, (2013). URL: http://prl.aps.org/abstract/PRL/v111/i15/e150404
[3] Berger, M.A., “Introduction to magnetic helicity”, Plasma Phys. Control Fusion 41, B167-B175 (1999). URL: http://iopscience.iop.org/0741-3335/41/12B/312
[4] Moffat, H.K., “Degree of knottedness of tangled vortex lines” J. Fluid Mech. 35, 117-129 (1969). URL: http://journals.cambridge.org/action/displayAbstract?fromPage=online&aid=382055&fulltextType=RA&fileId=S0022112069000991
[5] Kleckner, D. and Irvine, W.T.M. “Creation and dynamics of knotted vortices”, Nature Physics 9, 253-258 (2013). URL: http://www.nature.com/nphys/journal/v9/n4/full/nphys2560.html