Sergey A. Suslov
Swinburne University of Technology
Hawthorn, Victoria, Australia
Aleksandra A. Bozhko, Aleksander S. Sidorov, Gennady F. Putin
Perm State National Research University
Perm, Russia
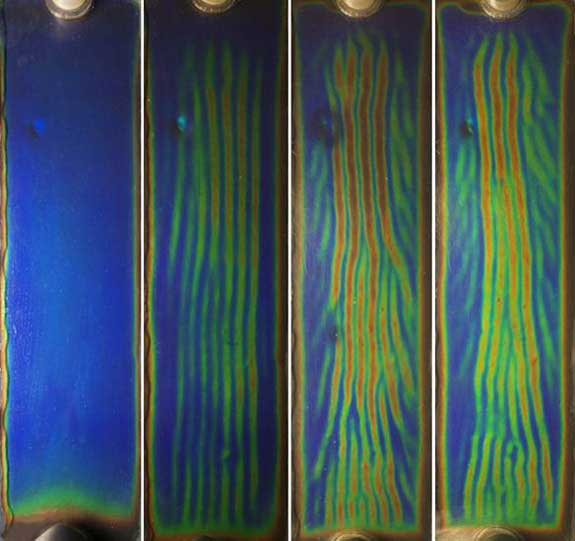
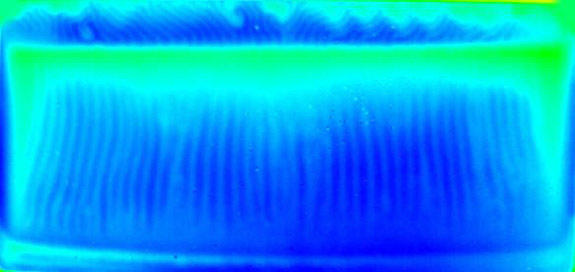
A ferrofluid is a liquid that becomes strongly magnetized in the presence of a magnetic field. The images above show the process of thermomagnetic convection in a ferrofluid, in which both magnetization and temperature play a part in the flow of the liquid.
Thermomagnetic convection arises due to the fact that the fluid's magnetization is sensitive to temperature. Cooler fluid is magnetized stronger, screening the external magnetic field more than warmer fluid. Subsequently, a more strongly magnetized cooler fluid is drawn to warmer regions with a stronger magnetic field.
Figure 1 is a composite of four photographs, showing a series of thermomagnetic convection patterns arising in a homogeneous ferrofluid heated from the front of the photographed surface. The average temperature gradient across the layer of fluid is fixed, but the magnetic field, applied normally to the image plane, increases from image to image. In the leftmost panel, with the weakest magnetic field, a vortical flow appears around the perimeter of the container. An increased magnetic field leads to the stationary thermomagnetic rolls apparent in the middle left pane. Finally, the strongest magnetic field causes the unsteady wave-like patterns in the two rightmost images. Flow visualization is performed using a heat-sensitive liquid crystal film glued on the surface of the layer facing the camera. This film reveals the surface temperature fields associated with the various flow patterns.
Figure 2 is an infrared photograph of two-cell thermomagnetic convection patterns arising in an undivided layer of a stratified ferrofluid that is being cooled from the front of the photographed surface. The vertical stratification is due to a slow gravitational sedimentation of magnetic nanoparticles.
Reporters and Editors
This image can be freely reproduced with the accompanying credit: "Aleksandra A. Bozhko, Aleksander S. Sidorov, Gennady F. Putin (Perm State National Research University, Perm, Russia) and Sergey A. Suslov (Swinburne University of Technology, Hawthorn, Victoria, Australia)."
Related Author Talk at the 2012 DFD Meeting
G2.00009: New type of thermal waves in a vertical layer of magneto-polarizable nano-suspension: theory and experiment
Contact Information
Dr. Sergey A. Suslov
Senior Lecturer in Applied Mathematics,
Mathematics H38,
Swinburne University of Technology,
PO Box 218, Hawthorn,
Victoria 3122, Australia
Phone: +61-3-9214 5952, Fax: +61-3-9214 8264
http://www.swin.edu.au/mathematics/staff/Sergey-Suslov